From the May 2016 issue of GCM magazine:
Nitrogen fertilization of bermudagrass affects carbon dioxide emissions
Increases in carbon dioxide emissions consistently correlated with increases in nitrogen applications, giving turfgrass managers another reason to avoid overfertilization.
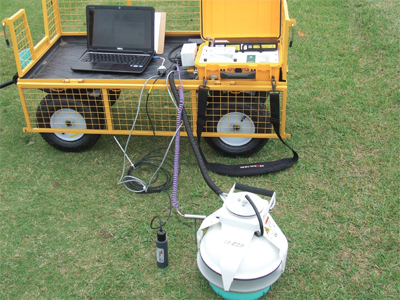
|
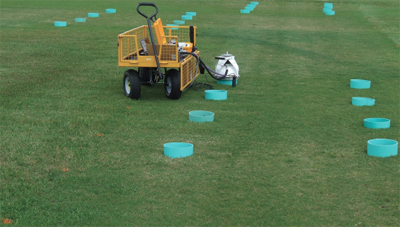 |
An automated CO2 flux infrared gas analyzer (IRGA) was used to measure CO2 flux from fertilized hybrid bermudagrass.
Photos by Said Hamido
|
Said A. Hamido, Ph.D.; Elizabeth Guertal, Ph.D., and C.W. Wood, Ph.D.
Read this story in GCM's digital edition »
Regardless of your thoughts about why it may be happening, atmospheric carbon dioxide (CO2) concentration has increased, from 280 ppmv (parts per million by volume) in 1850 to 400 ppmv in 2015 (5). We care about this fact because atmospheric CO2 is a factor in global air temperatures, which have been climbing by about 1.1 to 1.6 F (0.6 to 0.9 C) since 1750 (6). Soil and the vegetation associated with that soil can have profound effects on soil carbon and soil CO2 flux (“flux” is the transfer of carbon from one pool to another — such as from soil carbon to air — as CO2). Carbon storage in turfgrass systems and the underlying soil may serve as a carbon sink, storing significant amounts of carbon and thus helping mitigate climate change. Conversely, the turfgrass system may serve as a carbon source, emitting carbon emissions into the atmosphere. Are golf course fairways a carbon sink, or a source? The answer to this question is a product of many things, but maintenance practices such as fertilization, irrigation, mowing and use of fuel-powered equipment certainly influence that carbon flux (3).
Carbon dioxide flux has been studied less in turfgrass than it has in other land management systems. It is an area of interest because turfgrass is novel in its cultivation when compared with agricultural and forestry crops, as turfgrass is managed as a long-term perennial and vegetative crop, and also because the systems are high in carbon via thatch, shoots, underground stems such as rhizomes and stolons, and extensive root systems. Some studies with cool-season turfgrasses have indicated that golf course soils and home lawn soils sequester carbon, but such carbon storage was offset by carbon emissions from fuel combustion, nitrogen fertilizer and irrigation (7,8).
Because the little turfgrass carbon work that exists was performed on cool-season grasses, our research objective was to examine CO2 flux from an established sward of Tifway hybrid bermudagrass that had received five years of nitrogen fertilization at varying nitrogen rates.
Materials and methods
Figure 1. Carbon dioxide emissions from Tifway hybrid bermudagrass as affected by soil water content and nitrogen rate, 2012.
The study was initiated in March 2012 on an 8-year-old stand of Tifway hybrid bermudagrass (Cynodon dactylon × C. transvaalensis) located at the Auburn University Turfgrass Research Unit in Auburn, Ala., on a native soil (Marvyn loamy sand). The research plot area was managed as a golf course fairway, with mowing three of every seven days at a height of 1 inch (2.5 cm) and clippings not removed. Supplemental irrigation was provided in the absence of rainfall so that irrigation plus precipitation equaled 1 inch per week. The experimental design was a randomized complete block with four nitrogen rates of 0.5, 1, 2 and 4 pounds nitrogen/1,000 square feet/month for five months (24, 49, 98 and 196 kilograms nitrogen/hectare/year). All treatments were replicated three times. During the study, nitrogen was applied as urea (46-0-0) approximately monthly at the beginning of May, June, July, August and September. These nitrogen fertilization events corresponded to measurement days at around 120 (May) to 250 (September) days after the start of data collection. The same nitrogen rates had also been applied for five years to the same plots before the start of this experiment.
Carbon dioxide flux was measured weekly for 95 weeks, from March 2012 to December 2013 (2), using an automated CO2 flux infrared gas analyzer (IRGA). This system used a 7.8-inch-diameter (20-cm-diameter) PVC collar that was inserted 1.2 inches (3 cm) deep into each plot, with two collars placed in each plot. The IRGA was positioned over each collar, and CO2 flux was measured for a total of 24 measurements at each sampling (12 plots [4 nitrogen rates × 3 replications] × 2 subsamples per plot). Sampling always occurred between 10 a.m. and noon. Soil temperature and soil moisture were also collected every time CO2 flux was measured.
Seasonal CO2 fluxes
In summer of both years (June to August), soil CO2 flux was significantly correlated with nitrogen rate. As nitrogen rate increased, average flux of CO2 also increased, from 356.87 to 485.34 pounds CO2/acre/day (400 to 544 kilograms/hectare/day) from the lowest to highest nitrogen rate. There was variation from summer to summer, and CO2 flux was lower in 2013, when soil temperatures were not as high as those measured in 2012. There was also a spike in CO2 evolution, with the highest values recorded at two to three days after nitrogen fertilization. These recorded CO2 levels matched those previously measured for prairie soils (523.70 pounds CO2/acre/day [587 kilograms/hectare/day]), which were some of the first measured from a system that is somewhat similar to turfgrass (4).
Carbon dioxide flux in fall (September to November) and winter (December to February) was still significantly correlated with nitrogen rate (higher flux as nitrogen rate increased) in these sampling periods, even though the last nitrogen application had been made in the first week of September. Carbon dioxide fluxes were lowest in these sampling periods, a result of bermudagrass dormancy and falling soil temperatures. The lowest CO2 flux for each year was measured in late December, with values of 53 to 63 pounds CO2/acre/day (59 to 71 kilograms/hectare/day) from lowest to highest nitrogen rate, respectively. As the soil warmed in spring, CO2 flux once again increased, with values similar to those measured in summer, and, once again, CO2 flux increased as nitrogen rate increased. So, regardless of the time of year, CO2 flux was always greater from plots that had received a higher rate of nitrogen fertilizer.
In general, as nitrogen rate increased from 0.5 to 4 pounds nitrogen/1,000 square feet/month (24 to 196 kilograms nitrogen/hectare/month), CO2 flux increased from 288 to 379 pounds/acre/day (323 to 426 kilograms/hectare/day) in 2012, and from 226 to 315 pounds/acre/day (254 to 354 kilograms/hectare/day) in 2013. After every nitrogen application, there was an increase in CO2 emitted, but these increases were of short duration, and CO2 fluxes returned to typical values (as measured throughout each month) within two weeks after nitrogen fertilization. In the week after nitrogen fertilization, CO2 fluxes were usually about 25% higher than those measured in the third and fourth weeks after fertilization.
Annual cumulative fluxes of CO2
Total CO2 loss was different depending on the year, with higher emissions in 2012 than in 2013. This was likely a result of higher temperatures in 2012, especially in the summer. In each year, the total amount of CO2 emitted ranged from 40.14 to 71.37 tons/acre/year (90 to 160 Mg [megagrams])/hectare/year), averaged across the year. This compares favorably with another study that examined CO2 emissions from cool-season turfgrass (mixed Kentucky bluegrass/annual bluegrass/red fescue) with cumulative CO2 emissions of 44.60 tons/acre/year (100 Mg/hectare/year) (1).
In that cool-season study, mowing frequency was a treatment variable, as was removing or returning clippings. Plots that were mowed more frequently had greater CO2 emissions than those that were mowed infrequently (only once or three times from May to November). Another treatment in that cool-season study was clipping removal, and neither returning or removing clippings had an effect on CO2 emissions (1). Our study had frequent mowing (three times/week) and the clippings were left, both actions that could stimulate CO2 flux.
Soil moisture also affected CO2 flux. As Figure 1 shows, CO2 emissions increased as soil moisture content increased. The differences in emission rate caused by nitrogen rate are also shown in this graph, with CO2 emissions increasing as nitrogen rate increases.
What does this all mean?
Average daily CO2 flux and total cumulative CO2 emissions were always correlated with nitrogen fertilizer rate. As nitrogen rate increased, CO2 flux and emissions also increased, and these relationships were statistically significant and observed throughout the year, even when temperatures were low and bermudagrass was dormant. There were consistent and slight increases in CO2 flux for the week after each nitrogen fertilization, but values dropped again in later weeks after each fertilization. The upper nitrogen rates where CO2 flux was greatest are often considered high for a single application of a soluble fertilizer for hybrid bermudagrass managed as a fairway (clippings returned) (2 or 4 pounds nitrogen/1,000 square feet [98 or 196 kilograms nitrogen/hectare]). Carbon dioxide flux was lower at agronomically and environmentally sound rates (0.5 or 1 pound nitrogen/1,000 square feet [24 or 49 kilograms nitrogen/hectare]).
Of course, the question remains: Why do we care? Establishing the relationship between CO2 emissions and nitrogen fertilization of turfgrass helps us to determine the role of turfgrass in carbon cycling, and we currently lack much of that information for various turfgrass species and management practices. Carbon storage and the ability of plants and soils to sequester carbon are valuable in the effort to help reduce CO2 emissions. Demonstrating the usefulness of turfgrasses for carbon sequestration is important, because the ability of turfgrass and its underlying soil to serve as a carbon sink (storage) could be offset by CO2 emissions that are increased by practices such as fertilization and mowing (3). Ultimately, turfgrass managers need to know how their management practices affect the environmentally beneficial process of carbon sequestration. Properly fertilized and managed, turfgrasses and their underlying soil may turn out to be a significant carbon source. This work demonstrates that overfertilization of nitrogen negatively affects that carbon storage — an addition to the list of reasons not to apply excessive nitrogen.
Literature cited
- Allaire, S.E., C. Dufour-L’Arrivee, J.A. Lafond, R. Lalancette and J. Brodeur. 2008. Carbon dioxide emissions by urban turfgrass areas. Canadian Journal of Soil Science 88(4):529-532.
- Hamido, S.A., C.W. Wood and E.A. Guertal. 2016. Carbon dioxide flux from bermudagrass turf as affected by nitrogen rate. Agronomy Journal 108:1-7.
- Kong, L., Z. Shi and L.M. Chu. 2014. Carbon emission and sequestration of urban turfgrass systems in Hong Kong. Science of the Total Environment 473-474:132-138.
- Mielnick, P.C., and W.A. Dugas. 2000. Soil CO2 flux in a tallgrass prairie. Soil Biology & Biochemistry 32:221-228.
- National Oceanic and Atmospheric Administration. 2015. NOAA CO2 data for the Mauna Loa Observatory. NOAA. (http://co2now.org/images/stories/data/co2-mlo-monthly-noaa-esrl.pdf). Accessed Sept. 24, 2015.
- National Oceanic and Atmospheric Administration, National Climatic Data Center. 2012. Global Analysis - Annual 2012. NOAA. (www.ncdc.noaa.gov). Accessed April 10, 2013.
- Selhorst, A., and R. Lal. 2011. Carbon budgeting in golf course soils of Central Ohio. Urban Ecosystems 14:771-781.
- Selhorst, A., and R. Lal. 2013. Net carbon sequestration potential and emissions in home lawn turfgrass of the United States. Environmental Management 51:198-208.
Said A. Hamido is a postdoctoral Research Associate at the University of Florida’s Southwest Florida Research and Education Center in Immokalee, Fla. Elizabeth Guertal is a professor in the Department of Crop, Soil and Environmental Sciences at Auburn University, Auburn, Ala. C.W. Wood is professor emeritus at Auburn University and professor at and director of the University of Florida’s West Florida Research and Education Center in Jay, Fla., and Milton, Fla.